We take it for granted that virtually every habitat on Earth is alive with the sounds of animals, from the haunting songs of whales in the oceans to the riotous symphony of birds, frogs and insects in forests to the hubbub of humans and our technological creations in cities the world over. Yet for most of our planet’s history the only sounds were those of the wind, rain and waves.
In my work as a paleontologist, I seek to understand the lives of extinct animals—how they moved, what they ate, the sounds they might have made. I also serve as an animation and creature-design consultant for exhibits, television, movies and games. Among the most common topics I have been asked to tackle for these projects are those pertaining to animal sounds. Whether someone is reconstructing long-vanished pterosaurs for an academic study or designing a creature for a blockbuster film, sound is paramount in bringing both past and imaginary worlds to life.
Recent insights into the evolution of animal acoustics have led to a new understanding of how our modern-day soundscapes came to be. Fossils reveal when the major types of sound-production—and sound-detection—structures appeared in the forerunners of today’s invertebrate and vertebrate creatures. And in some cases, clever modeling has allowed scientists to re-create the ancient sounds themselves. Many details remain to be worked out, but we can now begin to piece together the dawn of the din.
Breaking the Silence
The fossil record indicates that life on Earth got its start 3.7 billion years ago. But those earliest organisms—including microbes and, much later, soft-bodied animals similar to today’s jellies—were a quiet bunch. It was not until the evolutionary burst that occurred in the Cambrian period, between 541 million and 485.4 million years ago, that animals acquired some basic sound-making behaviors related to locomotion and predation. Yet even then the hush under water, where these creatures lived, was probably punctuated only by the skittering of arthropod feet across sand or the faint grinding of a cephalopod breaking a shell. Meanwhile the terrestrial realm remained essentially silent. More than 200 million years passed before the buzzing of insects started to fill the air, giving rise to an entirely new acoustic world.
The oldest-known putative insect dates to 408 million years ago and was probably soundless and deaf. Scientists do not know exactly when insects started to first make or hear sounds, but the fossil record provides a minimum date: a katydid from around 250 million years ago has the sound-producing anatomy characteristic of this group. The earliest-known fossils of cicada relatives also date to this time. These insects can generate exceptionally loud sounds by rapidly buckling and unbuckling drumlike structures on their bodies called tymbals. The sound-production structures are so well preserved in some insect fossils that researchers can reconstruct the songs the creatures sang in life. In 2011 a team led by Jun-Jie Gu, now at Sichuan Agricultural University in China, worked out that a specific ancient katydid emitted songs tuned to a frequency of 6.4 kilohertz, which is roughly an octave higher than Mariah Carey’s highest recorded note.
For these earliest buzzing insects, the advantages of producing and hearing sound would have been numerous. They could communicate over distances, hear predators approach and perhaps even lure prey by mimicking the sounds of a target animal’s potential mate. Sound offered a new means of attracting mates and in so doing gave rise to a new kind of biological battle: the evolution of the loudest.
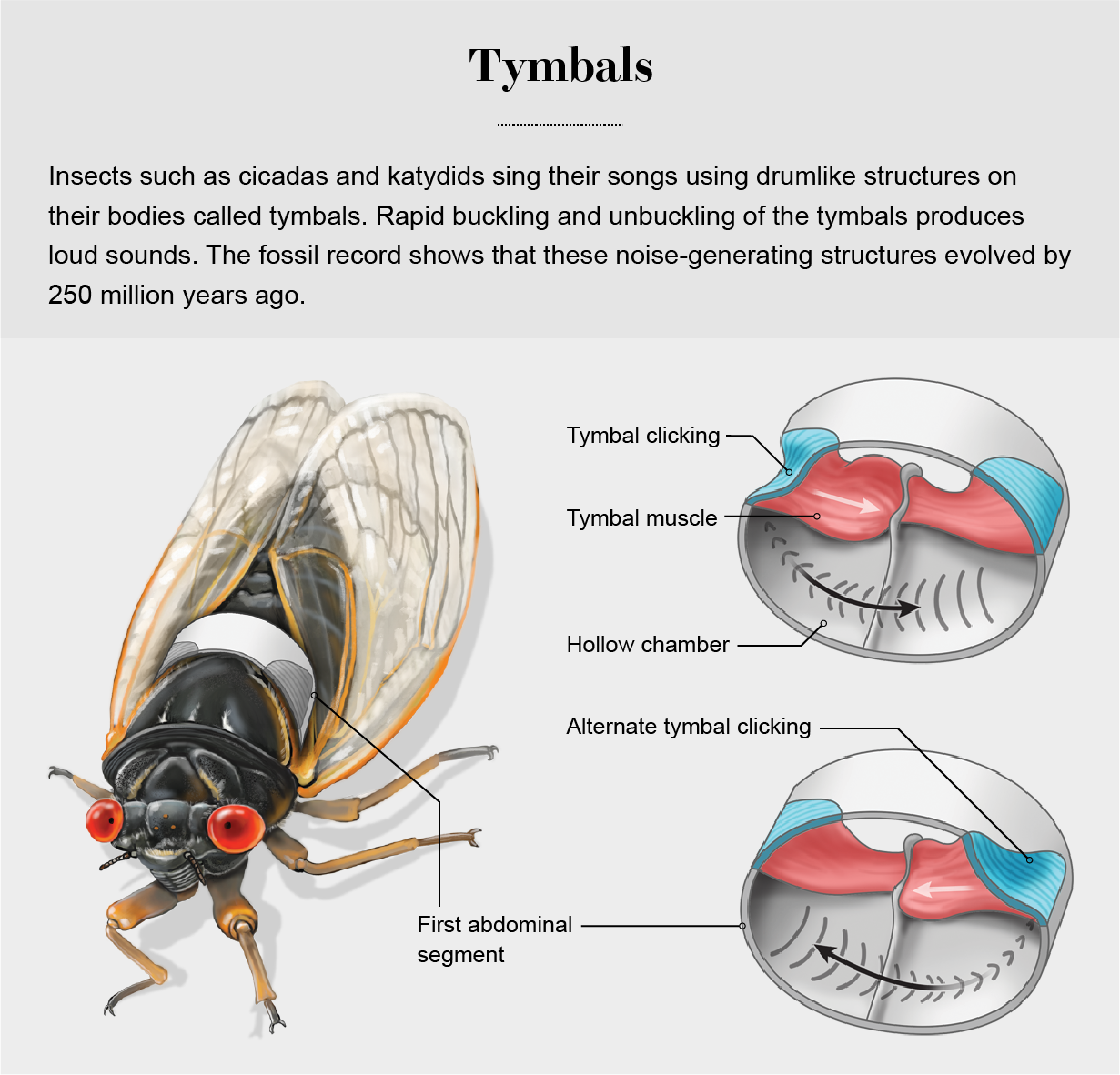
Vertebrates probably began experimenting with sound in some limited form around the same time that insects started buzzing and chirping. Today’s amphibians, reptiles and mammals all possess a larynx, or voice box, near the top of their airway. This fact suggests they inherited it from their last common ancestor, which would mean the larynx is nearly as old as land vertebrates themselves, going back 300 million years or so. It probably took millions of years for truly specialized or powerful vocalizations to evolve in these animals, however. Little is known about these early stages of vertebrate vocalization, not least because the larynx is made up of cartilage, which generally does not preserve well.
What we do know is that starting roughly 230 million years ago, during the Mesozoic era, vertebrate animals evolved a wide range of vocal abilities. That is when the world became truly loud. For example, frogs, with their rich repertoire of calls and songs, first showed up in the Mesozoic. Mammals also debuted around this time, and their characteristic chirps, growls and hisses probably evolved early on. Although we do not have much direct evidence of the sound-production apparatus in early mammals, we do have an exciting fossil record of their ears. The mammalian ear is unique, with three tiny bones in the middle ear, two of which are derived from bones that make up the jaw in most other vertebrates. These special ears are quite good at hearing high-frequency sounds, which could have helped the mammals find buzzing insect prey. These mammals might also have been capable of producing high-frequency sounds, allowing individuals to communicate with one another in a frequency range that few other animals could detect.
Among the most acoustically talented animals in the Mesozoic were the dinosaurs. In 1981 David Weishampel carried out one of the first reconstructions of a fossil animal’s vocalization, working with the herbivorous, duck-billed dinosaur Parasaurolophus. This creature had a giant crest on its head that connected to its airway. Weishampel demonstrated that the crest made for an excellent resonating chamber. By taking into account the size and shape of the crest, he was able to estimate the sound repertoire of Parasaurolophus and reproduce it with a model he built. (To this day, Weishampel affectionately refers to that rig as his “honker.”)
Increasingly sophisticated digital tools have made it possible to do this kind of in-depth analysis on many more specimens. Since 2008 Lawrence Witmer of Ohio University and his team have been using CT scans, along with fluid mechanics modeling, to assess sound production in various dinosaur species. They have discovered that the skulls of many dinosaurs contained complex chambers. Air flow through these spaces would have been correspondingly convoluted. The air flow helped to regulate body temperature, but it also might have enabled the animals to produce a wide array of sounds, including honks, bellows and trumpets.
Reconstruction of dinosaur vocalizations has long been a mainstay of the movie industry. The roars in films such as Jurassic Park are among the most famous portrayals of extinct-animal sounds. But Julia Clarke of the University of Texas at Austin and her colleagues found that the sounds of predatory dinosaurs such as Tyrannosaurus rex might have been more birdlike than mammal-like. Reporting on Clarke’s work, some journalists wrote the fearsome tyrannosaurs might have “honked” rather than “roared.” But before you begin picturing T. rex as a giant goose, it is worth noting the researchers meant “honk” from a biomechanics standpoint—a sound produced largely through the nose rather than the mouth, starting with the vibration of structures deep in the chest. Scaled to tyrannosaur size, that honk becomes a bass-baritone mega war trumpet. The beast could have sounded off with the full force of the entire brass section of the Los Angeles Philharmonic before entering battle armed with six-foot-long bone-crushing jaws.
Some dinosaurs were probably quite a bit less vocal than often portrayed, however. In the original Jurassic Park, the long-necked brachiosaurs trumpet like elephants. In reality, they were probably nearly voiceless. At most they might have been able to hiss. In all tetrapods—the group that includes the first land vertebrates and their descendants—the primary vocalizations in the larynx are controlled predominantly by the recurrent laryngeal nerve. This nerve always has the same strange configuration: it runs all the way down the neck, loops around large blood vessels in the upper and middle chest, and then runs back up the neck to the larynx. As a result, the signals for voice have to travel about twice the length of the neck.
For short-necked animals, including humans, the resulting delay in signal speed is trivial. But for a giant, long-necked dinosaur, this delay would be comparatively enormous—so much so, in fact, that there would be no way to properly control the rapid movement of the vocal cords during complex vocalizations such as honking or trumpeting. The next time you watch Jurassic Park, imagine honking hadrosaurs, trumpeting tyrannosaurs and hissing brachiosaurs.
In all likelihood, many dinosaurs were impressive vocalists. But one group in particular evolved some of the most sophisticated vocalizations of any animal. Members of this group still live among us: the birds. The earliest-known birds were identified from fossil deposits that are about 150 million years old, although their unique vocal abilities may have evolved somewhat later.
Birds have a special vocal structure called a syrinx. Their larynx is reduced, and the syrinx provides nearly all of their vocal control. Whereas the larynx resides at the top of the main airway, the syrinx sits at the bottom, where the trachea (aka the windpipe) branches out to the lungs. This anatomical arrangement offers several advantages. One of the most fundamental, described in 2019 by Tobias Riede of Midwestern University and his colleagues, is that it greatly improves the resonating efficiency. In other words, it produces more sound for the same energetic cost. Another advantage is that the syrinx can use the airstreams from the right and left lungs differently. Songbirds are particularly adept at this; the sounds from each side can be produced simultaneously or independently. In some cases, the two sides are specialized for different frequency ranges, enabling the bird to sing internal duets. The modern-day Hermit Thrush’s beautiful song results from this capability.
Scientists do not yet have a firm grasp on the origins of the syrinx. The oldest avian syrinx discovered to date comes from a specimen of the extinct bird Vegavis, described in 2016 by Clarke and her colleagues. Vegavis lived during the latest part of the Cretaceous period, 66 million to 69 million years ago. But its syrinx was already fairly specialized, with its expanded resonating space and asymmetry associated with dual-sided sound production. A more primitive version of the organ could have originated earlier.
Interestingly, the other vertebrate fliers of the Mesozoic, the pterosaurs, did not have a syrinx. So contrary to what Hollywood might have us believe, pterosaurs did not sound like birds. They probably sounded more like other reptiles. They might have growled, hissed, clicked or even bill clacked, opening and closing their beaks to make a clattering sound. The bill clack from a giant pterosaur, one with a nine-foot-long skull, could have been deafening to a wide range of creatures at close range.
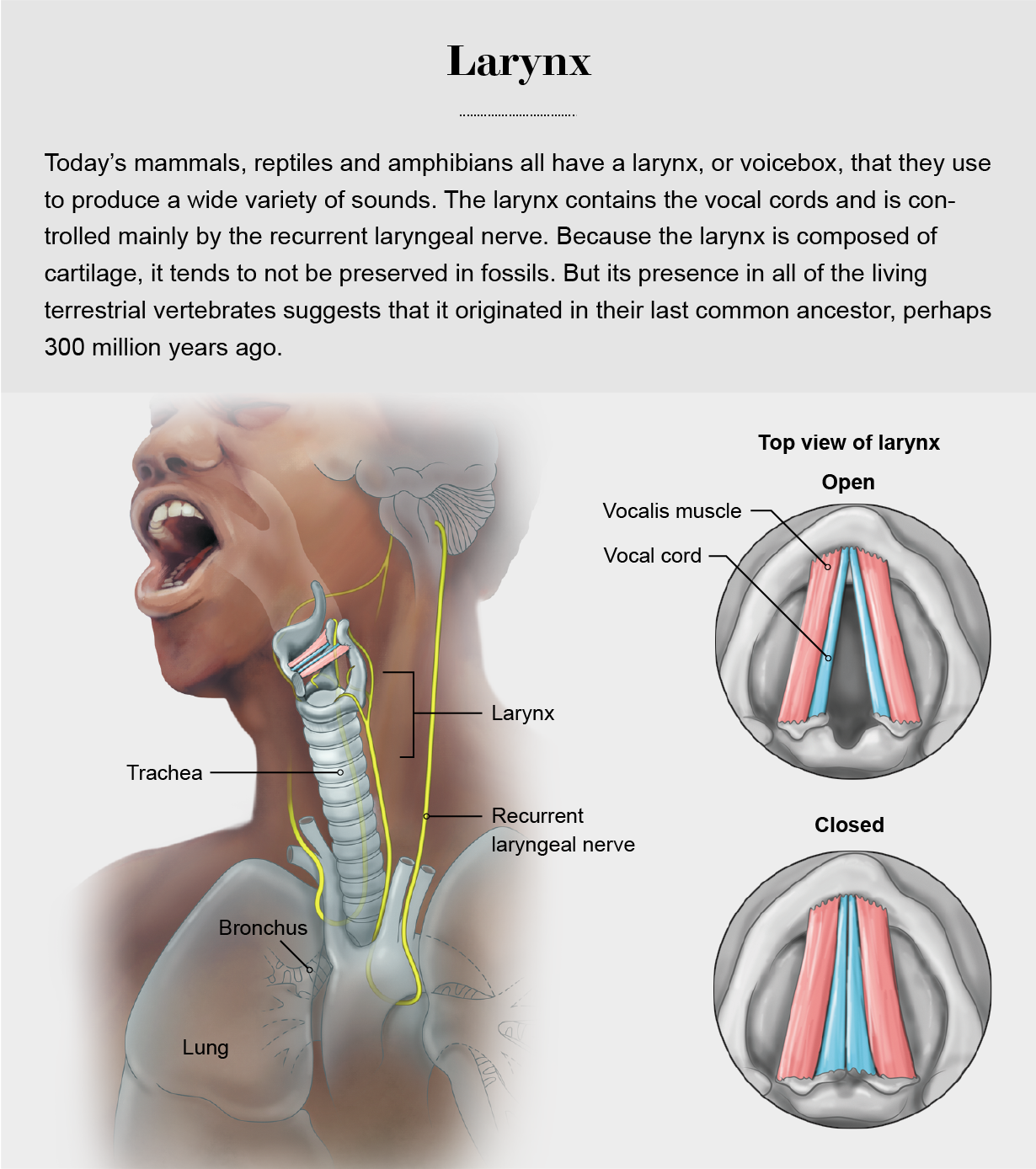
Seeing with Sound
With the arrival of the Cenozoic era, starting 66 million years ago, a whole new way of experiencing the world acoustically emerged: echolocation. Bats and whales appeared early in the Cenozoic, and both groups evolved this ability to see with sound. Echolocation requires quite a bit of sophisticated anatomy, though. To use echolocation, an animal has to produce a beam of sound, aim it in the desired direction, listen for the echoes, and then process those echoes to determine the distance, speed and general shape of whatever object the sounds bounce back from. It is a great trick for navigating and hunting in dark skies or murky waters.
Just like with light, the size of the smallest object that can be seen with echolocation is equal to half the wavelength of the emitted beam. Sound waves are much longer than light waves, however, so to get a decent picture of the environment or to see tiny insects, the emitter must produce very high-frequency sounds, which have shorter wavelengths than low-frequency sounds do. In bats, these calls, which are produced by the larynx and tongue, are typically far too high for us to hear. Human hearing maxes out at about 20,000 hertz, whereas bat calls range from about 10,000 to a staggering 200,000 hertz. We only ever hear the bats’ lowest-frequency calls; they sound like high squeaks to us, but they are the equivalent of bass-baritone voices to them.
We know roughly when bats started echolocating because of characteristics of the ear anatomy in fossil bats. To hear the echoes of ultrasonic calls, bats need comparatively giant inner ears. The primary structure of hearing in the skull is called a cochlea. Both of your cochlea would fit on the face of a dime with room to spare. If your cochlea were the same relative size as a bat’s, each would be about the size of a golf ball. The 50-million-year-old Icaronycteris index from western Wyoming is one of the earliest-known bats, and it already had skull characteristics associated with ultrasonic echolocation in living bats.
The evolution of echolocation in bats marked a major ecological revolution: vertebrates could now hunt insects on the wing in total darkness. After 275 million years, flying insects had nowhere to hide. Bats flourished as a result of this innovation. Today they make up roughly 25 percent of mammal species, and although some bats rely solely on vision to hunt, most of them echolocate.
As well as echolocation works in aerial environments, aquatic habitats are in some ways even more amenable to the use of reflected sound to determine where objects are in space. Water carries sound energy with far less signal loss per unit distance, giving aquatic echolocators a longer range than their aerial counterparts. High-frequency sounds always have reduced range compared with low-frequency sounds in the same environment, but in the water some dolphins can manage to make out objects with echolocation from as far as 650 feet away. The aerial bats, in contrast, cannot locate large objects more than 160 to 300 feet away; the range is much shorter for tiny insect prey.
Marine dolphins, such as the familiar bottlenose dolphin, can use echolocation to get a “first look” at long range in dark or cloudy water. River dolphins, such as those that live in the Amazon River, make even greater use of echolocation. The environment of these cetaceans is exceptionally murky. The river dolphins have smaller eyes and thus reduced visual acuity compared with other cetaceans, and they seem to rely heavily on echolocation to navigate and find food.
Marine mammals hold other sound records, too. If producing sound that travels as far as possible is the name of the game rather than trying to hear the echoes, then the best option is to use very low-frequency sounds. Big animals in aquatic environments have the natural advantage here. It is no surprise, then, that the champions of massive sonic range are the giant baleen whales. With larynxes up to two feet long and calls so low a human cannot hear them, some of these whales sing songs that can carry for hundreds, possibly thousands, of miles. Between the bats and the whales, the world is even noisier than we humans may realize.
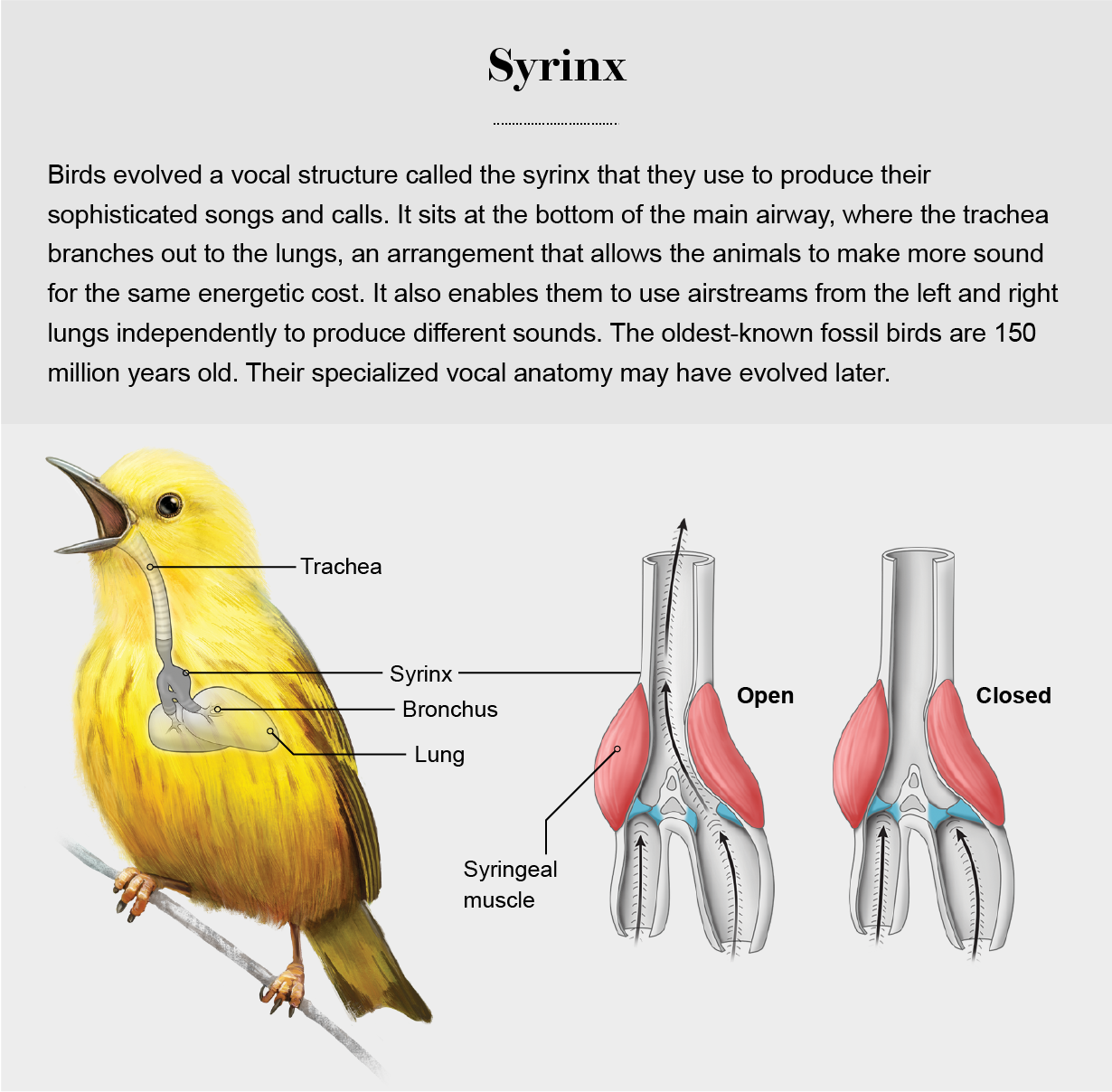
The Language Revolution
Some 230 million years after the sounds of early mammals began to permeate the world, vocalization took on a new role with the evolution of human language. The anatomical prerequisites for language, including a larynx that can adjust rapidly and sophisticated integration of the larynx and tongue, seem to date back at least to the origin of our genus, Homo. This means human ancestors may have had some form of speech as early as 2.8 million years ago. Exactly when and in which Homo species language first originated remains a matter of debate, though. Language arose not only from the anatomy that enables speech but also from the capacity for symbolic thought. Most models suggest Homo erectus, which debuted around 1.8 million years ago, was the first human ancestor to use symbols. But fully formed human language, with its complex rules of grammar and syntax, might be unique to our own species, which would mean it originated within the past few hundred thousand years.
It is not just the possession of language that is so powerful: humans are unique in their abilities to teach, learn and record language. Although researchers have successfully taught some other primates to use sign language, none of these educated apes has ever taught this language to others of its species, even when given the opportunity. In one case, a chimpanzee at the Duke University primate facility who had been taught sign language was reunited with his troupe. He tried to use his new skill set to communicate with his fellow chimps. After a week of attempts, his keepers found him in a corner of the paddock, where he had isolated himself. When they asked him in sign language why he was not with the other chimps, he signed back, “Because they are insects.”
Human language is one of the most impactful biological traits ever to have evolved. The formation of our social groups, societies and civilizations has been predicated on it. By coordinating our efforts through language, we have invented everything from agriculture to space shuttles—technologies that themselves contribute to contemporary soundscapes. Ours may not be the earliest animal sound, or the loudest, or the sweetest, but it is, in a sense, the one that most changed the world.